On the Production of Highest Energysolarprotons at 20 January 2005
On
January 20, 2005 NOAA reported an X7 importance flare with helio-coordinates
(14N, 61W), which started at 6:36 UT with maximal X-ray flux at 7:01 UT. The
associated CME had the largest sky-plane speed, exceeding 3000 km (Gopalswamy
et al., 2005). The first results on the unleashed Solar Energetic Proton (SEP)
event reported by space-born particle spectrometers (Mewaldt et al., 2005)
pointed to very hard energy spectra of accelerated protons. It stimulated
detailed investigation of the correspondent Ground Level Enhancement (GLE) #69,
having one of the goals to estimate the maximum energy of the solar
accelerators.
Available
theoretical and experimental data (from the huge GLR of 1956, see Fig.1,
unfortunately not well measured due to lack of appropriate detectors at this
time) on the GLEs confirm proton acceleration up to 20 GeV (Toptigin, 1983;
Dorman, 2004). The stochastic acceleration in the flares (Petrosian, 2006) and
shock acceleration in corona and interplanetary space (Gang and Zank, 2003) are
the two theories aimed to explain the origin and mechanisms of the particle
acceleration at the Sun. Middle and high-latitude neutron monitors cannot be
used for the reconstruction of the primary energy spectra above 5 GeV due to
very weak fluxes and relatively small sizes of the detectors.
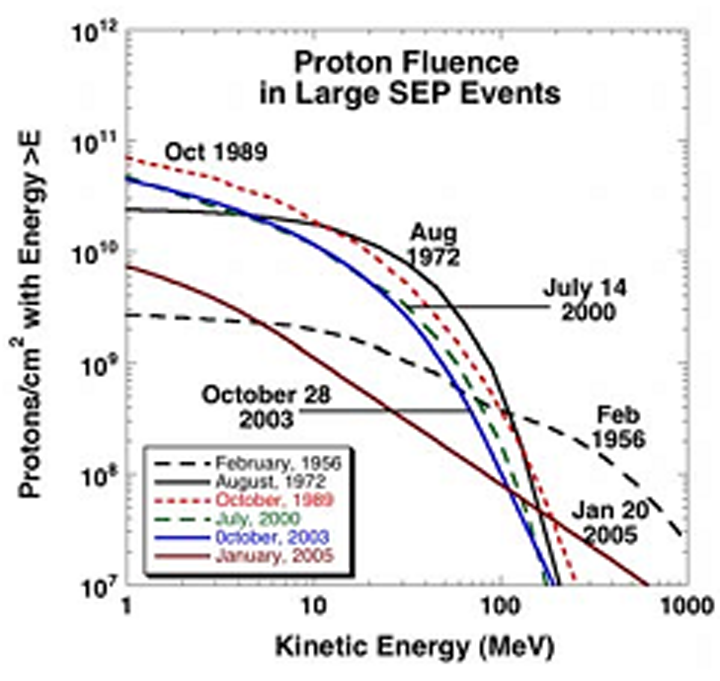
Figure 1.
Strongest SEP events of last 60 years
Therefore, recent years surface particle
detectors measuring Extensive Air Showers (EAS) were implemented for the
investigation of the highest energy solar protons and ions (Ryan, 1999; Ding,
2001; Poirier and D'Andrea, 2002; Chilingarian et al., 2003a). Due to their
large surface area and solid angle and high efficiency of the registration of
the charged particles, these detectors provide valuable information about the
solar proton fluxes well above 5 GeV.
The
Aragats Multidirectional Muon Monitor (AMMM, see Fig.2)a is located at
(40.25°N, 44.15°E, cutoff rigidity 7.6 GV) and on altitude 3200 m above sea
level; statistical accuracy of 3-min time series of is ≈0.17%, more sensitive than the neutron
monitor 18NM64, located at the same altitude.
The
AMMM consists of 45 (in 2006 enlarged to 100) plastic scintillators with
detecting surface of 1m2 and thickness of 5 cm each. The detector AMMM is located in the
underground hall of the ANI experiment (Chilingarian et al., 2003b) under 15 m
of soil and concrete, plus 12 cm. of iron bars. Only muons with energies larger
than 5 GeV can reach underground hall (muon traversal simulation in 2b), red
curve. 5 GeV muons are efficiently produced by primary protons of energy 35-50
GeV if we assume the power-law differential energy spectrum with spectral index
of g = -2.7 for Galactic Cosmic
Rays, and proton energies of 20-30 GeV if we assume spectral index g
= -4 to -5 (Chilingarian et al., 2005; Zazyan and Chilingarian, 2006).
During
GLE #69 on January 20, 2005 from 7:02 to 7:05 UT, AMMM detects a large flux
enhancement, see Fig. 2c.
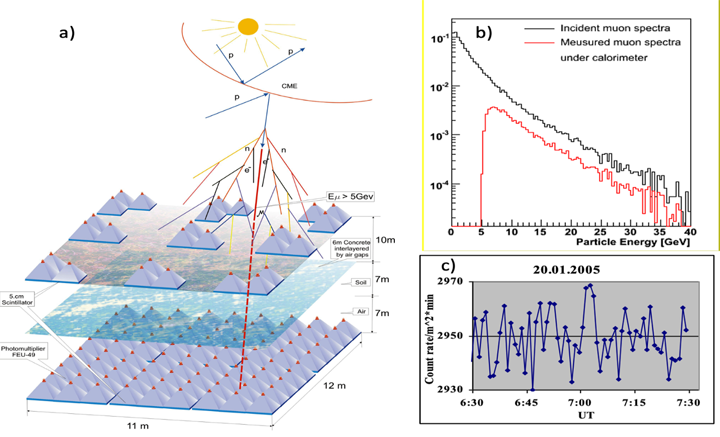
Figure 2.
a)Aragats Multidirectional Muon Monitor (AMMM); b) simulation of the muon flux
traversal; c)detection of flux enhancement during GLE N 69 (1-min time series).
We
compare AMMM observation other Aragats Space-Environmental Center (ASEC)
monitors (Chilingarian et al., 2006a) and other world-wide monitors, see
parameters of the monitors in Table 1, where types, heights, area, cutoff
rigidity and geographic coordinate of monitors are presented. Statistical
significance (for 3-min time series) is given for peaks occurred at 7:02 UT.
Table 3. Characteristics of the particle detectors
registered the GLE #69 at 20 January 2005
Detectors
|
Altitude(m) | Surface(m2) | Rigidity GV | Statistical significance | Geographic coordinate |
NANM 18NM64 | 2000 | 18 | 7.6 | 3.7 | 40.25oN, 44.15oE |
ANM 18NM64 | 3200 | 18 | 7.6 | 1.2 | 40.25oN, 44.15oE |
ASNT-8 channels | 3200 |
4 (60cm
thick)
4 (5cm thick)
|
7.6 |
0.2
1.5
|
40.25oN, 44.15oE |
AMMM | 3200 | 42 | 7.6 | 3.93 | 40.25oN, 44.15oE |
CARPET/Baksan | 1700 | 196 | 5.7 | 19 | 43.28oN, 42.69oE |
Tibet YBJ NM 28NM64 | 4300 | 28 | 14.1 | 12 | 30.11oN, 90.53oE |
Enhancement
of the count rate was seen from 7:02 till 7:04 UT with maximum at 7:03 UT.
Three out of the 45 one m2 scintillators of the AMMM were not operational at
the time, therefore only 42 m2 of muon detectors were in use to
measure the high energy muon flux. The estimated mean count rate of the
Galactic Cosmic Rays (GCR) as measured by the 42 m2 of the AMMM
detector is 123,818 particles per min. The additional signal at 7:03 UT equals
to 863 particles or enhancement of 0.70%. Taking into account that the standard
deviation of 1 min data is 352 (0.29%) the significance of the one-minute peak
at 7:03 UT was 2.5σ
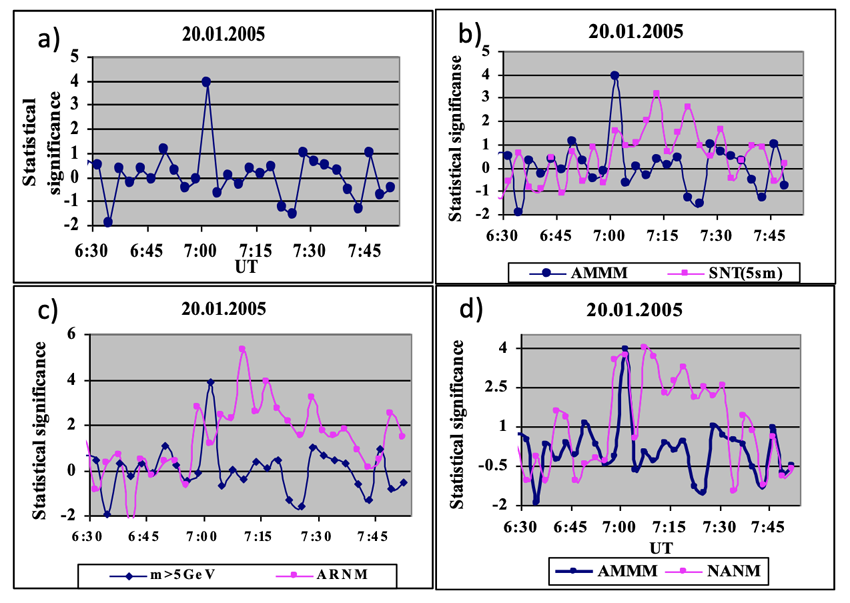
Figure 3.
Detection of the GLE 69 by different Aragats detectors
To
emphasize the peak in the AMMM time series we group the 1 min date in 3-min
time-intervals (see Fig. 3a). The mean count rate of GCR equals 371,454
particles per 3 min. The additional signal at 7:02 equals 2394 or enhancement
of 0.644%. If we adopt the Poisson standard deviation for the 3-min time series
0.164% (see detailed discussion on the determination of the significance of
detected enhancement in Chilingarian et al., 2006b) we come to the significance
of 3.93 for the 3 min peak at 7:02-7:05 UT. The excess count rate registered at
AMMM during the interval 7:02-7:05 UT corresponds to the flux (3.1 ± 0.8) · 10-5 muons/cm2/s.
In
Figs. 3c and 3d the count rate enhancements measured by the Aragats Neutron
Monitor (ANM), located at 3200 m ASL and Nor-Amberd Neutron Monitor (NANM)
located at 2000 m ASL are presented (both neutron monitors are 18NM64 type).
From the figures we can see that the enhancement at the neutron monitors
started ≈3 min earlier than the peak detected by the AMMM and in the interval
6:59-7:45 both ANM and NANM show at least two peaks having significance higher
than 3σ. The 5 cm thick plastic
scintillators of upper layer of the Aragats Solar Neutron Telescope (ASNT) is
sensitive to charged particles with energies greater than 7 MeV. As we can see
in Fig. 3b at the same time 6:59-7:45 ASNT also detect several significant peaks.
Analogous patterns were detected by the neutron monitors from the world- wide
network (Flueckiger et al., 2005).
The energies of the primary
solar protons giving rise to the secondary neutrons (registered by the neutron
monitors) and low energy charged particles (registered by surface scintillator
detectors) are smaller than the energies of the primary proton that create the
5 GeV muons in the atmosphere. Therefore, we conclude that maximal solar proton
energy at 7:12-7:45 was less comparing with 7:02-7:05 when pronounced peak in
>5 GeV muon time series was detected. Of course, absence of signal in the
AMMM also can be due anisotropic solar protons flux. However, despite the 20
January event was extremely anisotropic at the GLE onset, very soon after onset
solar proton flux became rather isotropic (Plainaki et al., 2007; Moraal et
al., 2005).
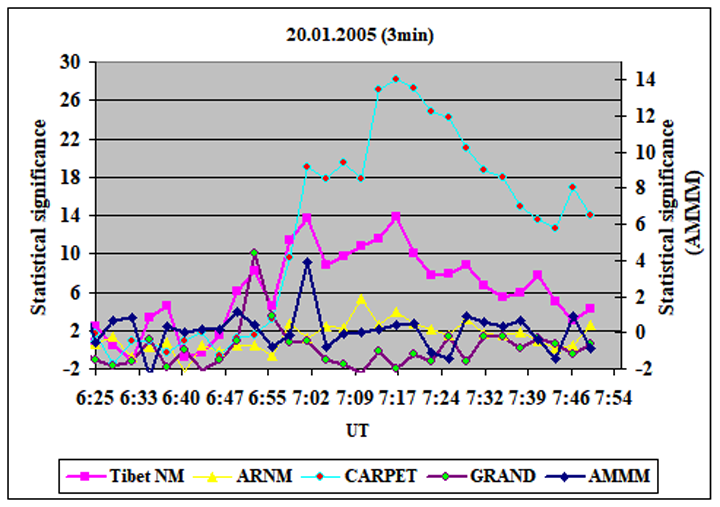
Figure 4.
Comparison of the time series of the particle detector sensitive to the highest
energies of solar particles: CARPET (energy range >6 GeV), Tibet NM (>13
GeV) and AMMM (>20 GeV).
The
20 January GLE was detected by several EAS detectors, measuring shower charge
particles (mostly muons and electrons) (D'Andrea and Poirier, 2005; Ryan, 2005)
and by Tibet YBJ neutron monitor (Miyasaka, 2005); all ensuring registration of
highest primary proton energies of 10- 15 GeV.
We
can see in Fig. 4 rather good agreement of the time series profiles. CARPET and
YBJ NM demonstrate high significance peaks in the same time at 7:02. Smaller
significance values of AMMM comparing with CARPET and YBJ NM is explained by
the much higher threshold of AMMM and large index of the proton flux energy
spectrum g= 4-5 (Bieber et al., 2005;
Miyasaka, 2005).
Bieber,
J., Clem, J., Evenson, P., et al. Largest GLE in half a century: neutron
monitor observations of the January 20, 2005 event, in: Proceedings of the 29th
International Cosmic Ray Conference (Pune), vol. 1, pp. 237-240, 2005.
Chilingarian,
A., Babayan, V., Bostandjyan, N., et al. Monitoring and forecasting of the
geomagnetic and radiation storms during the 23rd solar cycle: Aragats Regional
Space Whether Center. Adv. Space Res. 31, 861-865, 2003a.
Chilingarian,
A., Avakyan, K., Babayan, V., et al. Aragats Space- Environmental Center:
status and SEP forecasting possibilities. J. Phys. G Nucl. Partic. Phys. 29,
939-952, 2003b.
Chilingarian,
A., Arakelyan, K., Avakyan, K., et al. Correlated measure- ments of secondary
cosmic ray fluxes by the Aragats Space Environ- mental Center monitors. NIM
A543, 483-496, 2005.
Chilingarian,
A., Gharagyozyan, G., Karapetyan, G., et al. Statistical methods for signal
estimation of point sources of cosmic rays. Astropart. Phys. 25, 269-276,
2006a.
Chilingarian,
A., Bostandjyan, N., Eganov, V.S., et al. On the highest energies of proton
acceleration at the Sun on January, in: Proceedings of the 2nd International
Symposium on Solar Extreme Events, Nor- Amberd, Armenia, TIGRAN METZ, pp.
180-185, 2006b.
D'Andrea,
C., Poirier, J. Ground level muons coincident with the 20 January 2005 solar
flare. Geophys. Res. Lett. 32, L14102, doi:10.1029/ 2005GL023336, 2005.
Ding,
L. Variations in cosmic ray intensity observed with the L3 + cos- mics shower
array detectors and the intense solar flare on 14 July 2000, in: 27th ICRC,
SH1.07, 3372. Hamburg, 2001.
Dorman,
L.I. Cosmic Rays in the Earth's Atmosphere and Underground. Kluwer Academic
Publishers, P. 3, 2004.
Flueckiger,
H., Butikofer, R., Mozer, M.R., et al. The cosmic ray ground level enhancement
during the Forbush decrease in January 2005, in: Proceedings of the 29th
International Cosmic Ray Conference, vol. 1, pp. 225-228, 2005.
Gang,
L., Zank, G.P. Energetic particle acceleration and transport at coronal mass
ejection-driven shocks. J. Geophys. Res. 108, 1082, doi:10.1029/202JA009666,
2003.
Gopalswamy,
N., Xie, H., Yashiro, S., Usoskin I. Coronal mass ejections and ground level
enhancements, in: Proceedings of the 29th Interna- tional Cosmic Ray
Conference, vol. 1, pp. 169-173, 2005.
Mewaldt,
R.A., Looper, M.D., Cohen, C.M.S., et al. Solar-particle energy spectra during
the large events of October-November 2003 and January 2005, in: Proceedings of
the 29th International Cosmic Ray Conference (Pune), vol. 1, pp. 101-104, 2005.
Miyasaka,
H., and the YBJ NM collaboration. The Solar Event on 20 January 2005 observed
with the Tibet YBJ Neutron monitor obser- vatory. Official CD of 29th I.C.R.C.,
Pune, India, 2005.
Moraal,
H., McCracken, K.G., Schoeman, C.C., et al. The ground level enhancement of 20
January 2005 and 28 October 2003, in: Proceed- ings of the 29th International
Cosmic Ray Conference, vol. 1, pp. 221-224, 2005.
Petrosian,
V., Liu, S. Stochastic particle acceleration in solar flares, in: Proceedings
of the 2nd International Symposium on Solar Extreme Events, Nor-Amberd,
Armenia, TIGRAN METZ, pp. 3-19, 2006.
Plainaki,
C., Belov, A., Eroshenko, E., et al. Modeling ground level enchancements: the
event of 20 January 2005. J. Geophys. Res. 112, doi:10.1029/2006JA011926, 2007.
Poirier,
J., D'Andrea, C. Ground level muons in coincidence with the solar flare of
April 15, 2001. Geophys. Res. 107 (A11), 1376, doi:10.1029/ 2001JA009187, 2002.
Ryan,
J.M. for Milagro collaboration. Detection of 6 November 1997 ground level event
by Milagrito, in: 26th ICRC, Salt Lake City 6, p. 378, 1999.
Ryan,
J.M., and the Milagro Collaboration. Ground-level events mea- sured with
Milagro, in: Proceedings of the 29th International Cosmic Ray Conference, vol.
1, pp. 245-248, 2005.
Toptigin,
I.N. Cosmic rays in the interplanetary magnetic fields. Moscow, 108, in
Russian, 1983.
Zazyan,
M., Chilingarian, A. On the possibility to deduce solar proton energy spectrum
of the 20 January 2005 GLE using Aragats and Nor- Amberd neutron monitor data,
in: Proceedings of the 2nd Interna- tional Symposium on Solar Extreme Events,
Nor-Amberd, Armenia, TIGRAN METZ, pp. 200-202, 2006.